The Sun: Our Nearest Star and Life Sustainer
Introduction
The Sun, an immense sphere of hot plasma, is the central star of our solar system and the most crucial source of energy for life on Earth. This glowing celestial body has been the subject of human fascination and study for centuries, influencing cultures, religions, and sciences. Understanding the Sun is essential for comprehending not only our place in the cosmos but also the fundamental processes that sustain life on our planet.
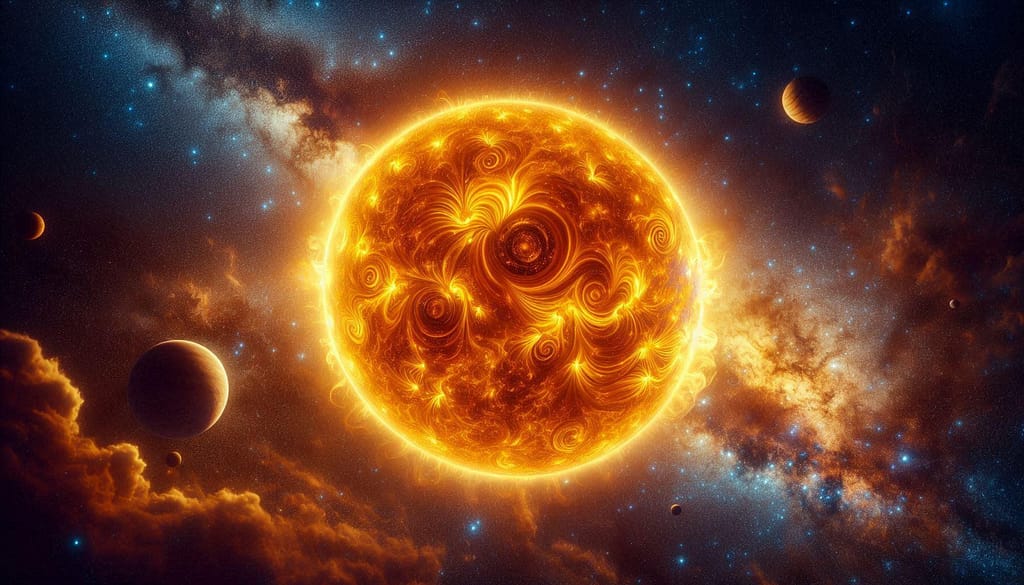
key characteristics of the Sun:
Characteristic | Description |
---|---|
Type | G-type main-sequence star (G2V) |
Age | Approximately 4.6 billion years |
Diameter | About 1.39 million kilometers (864,000 miles) |
Radius | About 696,340 kilometers (432,685 miles) |
Mass | 1.989 x 10^30 kilograms (333,000 times Earth) |
Volume | Approximately 1.41 x 10^18 cubic kilometers |
Surface Area | About 6.09 x 10^12 square kilometers |
Composition | ~74% hydrogen, ~24% helium, ~2% other elements |
Core Temperature | About 15 million degrees Celsius (27 million degrees Fahrenheit) |
Surface Temperature | About 5,500 degrees Celsius (9,932 degrees Fahrenheit) |
Photosphere Temperature | About 5,500 degrees Celsius |
Chromosphere Temperature | Ranges from 4,000 to 25,000 degrees Celsius |
Corona Temperature | Up to several million degrees Celsius |
Luminosity | 3.828 x 10^26 watts |
Energy Source | Nuclear fusion (proton-proton chain reaction) |
Rotation Period (Equator) | About 25 days |
Rotation Period (Poles) | About 35 days |
Sunspot Cycle | Approximately 11 years |
Distance from Earth | Approximately 149.6 million kilometers (1 AU) |
The Size and Rotation of the Sun
Size of the Sun
The Sun is an enormous celestial body, dwarfing the planets in our solar system. Its size can be described in terms of several key measurements:
- Diameter: The Sun has a diameter of approximately 1.39 million kilometers (864,000 miles). This is about 109 times the diameter of Earth.
- Volume: Given its vast diameter, the Sun’s volume is colossal, capable of holding about 1.3 million Earths inside it.
- Mass: The Sun’s mass is around 1.989 x 10^30 kilograms, which is 333,000 times the mass of Earth. This massive quantity of matter accounts for about 99.86% of the total mass of the entire solar system.
- Surface Area: The surface area of the Sun is about 6.09 x 10^12 square kilometers, over 11,990 times the surface area of Earth.
The Sun’s immense size plays a critical role in its ability to generate and radiate energy through nuclear fusion. This process occurs in the core, where the pressure and temperature are sufficiently high to fuse hydrogen atoms into helium, releasing vast amounts of energy.
Rotation of the Sun
Unlike Earth, the Sun is not a solid body; it is composed of hot plasma. This composition results in differential rotation, meaning different parts of the Sun rotate at different speeds.
- Equatorial Rotation: At the equator, the Sun rotates approximately once every 25 days.
- Polar Rotation: Near the poles, the Sun rotates more slowly, taking about 35 days to complete one rotation.
This differential rotation is due to the Sun’s gaseous state, allowing the equatorial regions to spin faster than the polar regions. The varying rotation speeds cause the Sun’s magnetic field to twist and warp, leading to the formation of sunspots, solar flares, and other magnetic phenomena.
Measuring Solar Rotation
Solar rotation is measured through several methods, including:
- Sunspots Tracking: Observing and tracking the movement of sunspots across the Sun’s surface provides a visible indication of its rotation.
- Doppler Shift: By measuring the Doppler shift of spectral lines in the light emitted by the Sun, scientists can determine the rotational velocity of different parts of the Sun.
Effects of Solar Rotation
The Sun’s rotation has significant effects on its magnetic field and solar activity:
- Solar Cycle: The Sun experiences an approximately 11-year cycle of magnetic activity, driven by its differential rotation. This cycle includes periods of high and low sunspot activity, known as solar maximum and solar minimum.
- Solar Flares and CMEs: The twisting and tangling of the magnetic field due to differential rotation can lead to solar flares and coronal mass ejections (CMEs). These phenomena release vast amounts of energy and charged particles into space, which can impact Earth’s magnetosphere, satellites, and communication systems.
- Helioseismology: The study of oscillations and waves within the Sun, known as helioseismology, helps scientists understand its internal structure and dynamics, including rotation.
Conclusion
The Sun’s size and rotation are fundamental characteristics that influence its behavior and impact on the solar system. Its immense size enables it to generate the energy that sustains life on Earth, while its differential rotation drives the solar activity that affects space weather. Continued study of these aspects of the Sun enhances our understanding of stellar dynamics and prepares us for the challenges posed by solar phenomena.
Structure and Composition
The Sun is a G-type main-sequence star (G2V), primarily composed of hydrogen (about 74%) and helium (about 24%), with trace amounts of other elements like oxygen, carbon, neon, and iron. It has a complex structure consisting of several layers:
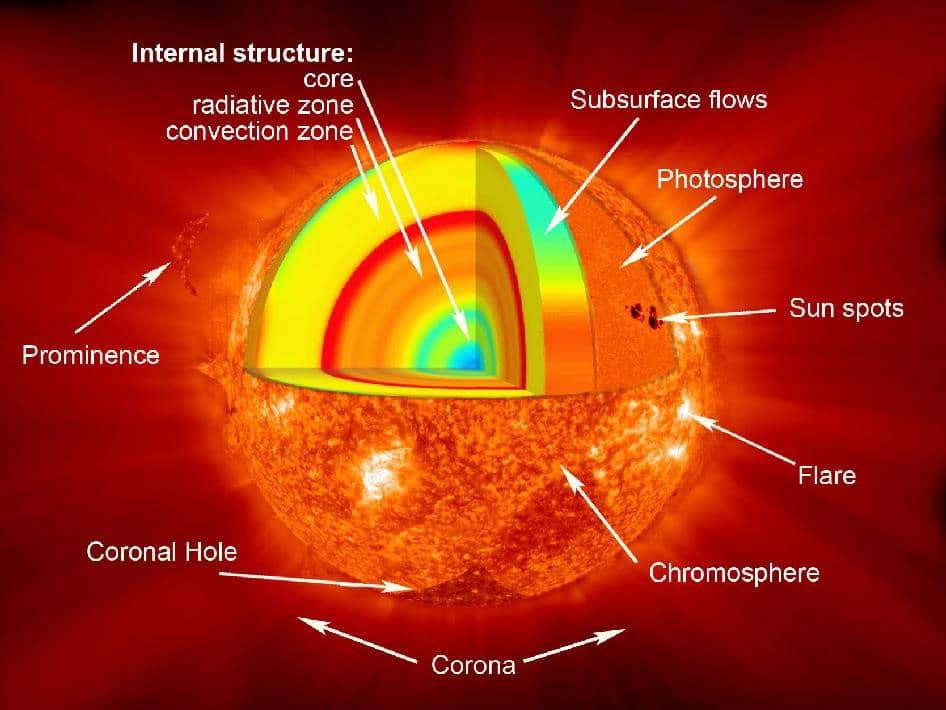
- Core: The core is where nuclear fusion occurs, converting hydrogen into helium and releasing immense amounts of energy. The temperature here reaches about 15 million degrees Celsius (27 million degrees Fahrenheit).
- Radiative Zone: Surrounding the core, the radiative zone extends outward to about 70% of the Sun’s radius. In this region, energy produced in the core slowly radiates outward through a process of photon absorption and re-emission.
- Convective Zone: Above the radiative zone, the convective zone spans the outer 30% of the Sun’s radius. Here, the temperature drops enough for the energy to be transported by convection currents—hot plasma rises, cools as it nears the surface, and then sinks back down to be reheated.
- Photosphere: The visible surface of the Sun, the photosphere, has a temperature of about 5,500 degrees Celsius (9,932 degrees Fahrenheit). It is here that sunlight is emitted, and sunspots—cooler, darker regions caused by magnetic activity—can be observed.
- Chromosphere: Above the photosphere lies the chromosphere, a layer that appears red during solar eclipses. It is a few thousand kilometers thick and has a temperature ranging from 4,000 to 25,000 degrees Celsius.
- Corona: The outermost part of the Sun’s atmosphere, the corona, extends millions of kilometers into space and is surprisingly hotter than the surface, with temperatures soaring up to several million degrees Celsius. The corona is visible during a total solar eclipse as a white halo around the Sun.
The Age of the Sun
Formation and Early Years
The Sun, like all stars, formed from a giant molecular cloud composed primarily of hydrogen and helium. Approximately 4.6 billion years ago, a disturbance—possibly a nearby supernova explosion—triggered the gravitational collapse of a region within this cloud. As the region collapsed, it began to spin and flatten into a rotating disk, with the central mass forming the protosun.
As the protosun accumulated more mass, the pressure and temperature in its core increased until nuclear fusion began, marking the birth of the Sun as a main-sequence star. This fusion process, where hydrogen atoms fuse to form helium, releases vast amounts of energy, causing the Sun to shine.
Current Age
The Sun is currently estimated to be about 4.6 billion years old. This estimation is based on various methods, including:
- Radiometric Dating: By dating the oldest meteorites found in the solar system, scientists have determined the age of the solar system, and consequently, the Sun, to be about 4.6 billion years. These meteorites formed from the same protoplanetary disk that gave birth to the Sun and planets.
- Stellar Models: Models of stellar evolution track the lifecycle of stars based on their mass and composition. By comparing these models to the current properties of the Sun, such as its luminosity, temperature, and chemical composition, astronomers can estimate its age.
- Helioseismology: The study of oscillations and waves within the Sun, known as helioseismology, provides insights into its internal structure and processes. This information helps refine models of the Sun’s evolution and age.
Lifecycle and Future
The Sun is currently in the middle of its life cycle, residing on the main sequence, where it has been for about 4.6 billion years. It has enough hydrogen in its core to continue nuclear fusion for roughly another 5 billion years.
Eventually, the Sun will exhaust its hydrogen fuel and undergo significant changes:
- Red Giant Phase: As hydrogen in the core depletes, the core will contract and heat up, causing the outer layers to expand and cool. The Sun will become a red giant, significantly larger and more luminous than it is now.
- Helium Fusion: In the red giant phase, helium fusion will begin in the core, producing carbon and oxygen.
- Planetary Nebula and White Dwarf: After shedding its outer layers, the remaining core will contract into a white dwarf—a hot, dense, Earth-sized remnant. This process will leave behind a beautiful planetary nebula, a shell of ionized gas glowing brightly.
Conclusion
The Sun, currently 4.6 billion years old, is a middle-aged star with a stable and predictable life cycle. Understanding its age and future helps us grasp not only the history and evolution of our solar system but also the broader processes governing stellar evolution in the universe. This knowledge allows scientists to predict the long-term fate of Earth and other planets in the solar system.
The Physics of the Sun
Nuclear Fusion
At the core of the Sun lies the process that powers it: nuclear fusion. This is where hydrogen nuclei (protons) fuse to form helium, releasing vast amounts of energy in the form of light and heat. The primary fusion process in the Sun is the proton-proton chain reaction, which can be summarized as follows:
- Proton-Proton Chain Reaction:
- Two protons (hydrogen nuclei) collide with sufficient energy to overcome their electrostatic repulsion and fuse, forming a deuterium nucleus (one proton and one neutron), a positron, and a neutrino.
- The deuterium nucleus then fuses with another proton to form helium-3, emitting a gamma ray.
- Two helium-3 nuclei collide to form helium-4, releasing two protons.
This series of reactions converts a small amount of mass into energy according to Einstein’s famous equation, ( E = mc^2 ).
Energy Transport
The energy produced in the Sun’s core travels outward through several layers before reaching the surface and radiating into space. This energy transfer occurs in two main regions: the radiative zone and the convective zone.
- Radiative Zone:
- Extending from the core to about 70% of the Sun’s radius, the radiative zone is where energy is transported by radiation. Photons are absorbed and re-emitted countless times, a process known as radiative diffusion. It can take millions of years for energy to travel through this region due to the dense plasma.
- Convective Zone:
- Beyond the radiative zone lies the convective zone, where the temperature is lower, allowing energy to be transported by convection. Hot plasma rises toward the surface, cools, and sinks back down to be reheated. This convective movement creates convection cells, observable on the surface as granules.
Solar Atmosphere
The Sun’s atmosphere consists of several layers, each with unique physical properties:
- Photosphere:
- The visible surface of the Sun, with a temperature of about 5,500 degrees Celsius. Sunspots, cooler regions caused by magnetic activity, are visible here.
- Chromosphere:
- Above the photosphere, the chromosphere has a temperature range from 4,000 to 25,000 degrees Celsius. It appears red during solar eclipses due to the emission of hydrogen-alpha light.
- Corona:
- The outermost layer, extending millions of kilometers into space, with temperatures soaring to several million degrees Celsius. The corona’s high temperature, compared to the surface, is a subject of ongoing research, involving processes like magnetic reconnection and wave heating.
Magnetic Fields and Solar Activity
The Sun’s magnetic field is generated by the dynamo effect in its convective zone. This field drives various solar phenomena:
- Sunspots:
- Temporary regions of strong magnetic activity, appearing darker due to lower temperatures.
- Solar Flares:
- Sudden, intense bursts of radiation resulting from the release of magnetic energy.
- Coronal Mass Ejections (CMEs):
- Massive bursts of solar wind and magnetic fields rising above the corona and being released into space. CMEs can impact Earth’s magnetosphere, causing geomagnetic storms.
Helioseismology
Helioseismology is the study of the Sun’s internal structure through its oscillations and waves. By observing the frequencies and modes of these oscillations, scientists can infer details about the Sun’s internal temperature, composition, and dynamics.
Conclusion
The Sun’s functioning involves complex and interconnected physical processes. From nuclear fusion at its core to the dynamic behavior of its magnetic field and the intricate layers of its atmosphere, the Sun’s physics are fundamental to our understanding of not only our star but stellar phenomena in general. Studying the Sun’s physics helps us predict solar activity, understand stellar life cycles, and grasp the fundamental processes that govern the universe.
The Sun’s Energy and Its Impact on Earth
The Sun’s energy, primarily in the form of light and heat, is fundamental to life on Earth. This energy drives the climate and weather, powers photosynthesis in plants, and influences ocean currents. Solar radiation also has both beneficial and harmful effects on human health and technology. While it provides Vitamin D and supports ecosystems, excessive exposure can lead to skin cancer and other health issues.
Solar Eclipse: A Celestial Phenomenon
What is a Solar Eclipse?
A solar eclipse occurs when the Moon passes between the Earth and the Sun, temporarily blocking the Sun’s light either partially or completely. This alignment casts a shadow on Earth and creates one of nature’s most spectacular visual phenomena.
Types of Solar Eclipses
There are three main types of solar eclipses:
- Total Solar Eclipse:
- In a total solar eclipse, the Moon completely covers the Sun, as viewed from Earth. This happens when the Moon is close enough to Earth in its orbit to appear larger than the Sun in the sky. The sky darkens as if it were night, and the Sun’s corona (the outer atmosphere) becomes visible as a stunning halo around the Moon. A total solar eclipse can only be seen from a narrow path on Earth’s surface, called the path of totality.
- Partial Solar Eclipse:
- During a partial solar eclipse, only a portion of the Sun is obscured by the Moon. This occurs when the Sun, Moon, and Earth are not perfectly aligned. Partial eclipses are more common than total eclipses and can be seen over a much larger area.
- Annular Solar Eclipse:
- An annular solar eclipse happens when the Moon is too far from Earth to completely cover the Sun. Instead, the Moon appears smaller, and a ring of the Sun’s surface, or “annulus,” remains visible around the Moon. This “ring of fire” creates a dramatic visual effect. Like total eclipses, annular eclipses are visible from a narrow path.
Phases of a Solar Eclipse
A solar eclipse progresses through several phases:
- First Contact:
- The Moon begins to move in front of the Sun, creating a small “bite” on the Sun’s edge.
- Second Contact:
- For total and annular eclipses, this marks the beginning of total or annular eclipse when the entire Sun is covered by the Moon except for the outer edges (for annular) or the corona (for total).
- Maximum Eclipse:
- The peak of the eclipse, where the maximum amount of the Sun is covered by the Moon.
- Third Contact:
- The end of the total or annular phase as the Moon starts to move away, allowing the Sun to reappear.
- Fourth Contact:
- The Moon completely exits the Sun’s disk, concluding the eclipse.
Viewing a Solar Eclipse
While solar eclipses offer incredible views, they must be observed with caution:
- Eye Safety:
- Never look directly at the Sun without proper eye protection, as it can cause permanent eye damage. Use eclipse glasses or a solar viewer that meets the ISO 12312-2 safety standard.
- During totality in a total solar eclipse, it is safe to look at the Sun with the naked eye, but only during the brief period when the Sun is completely covered by the Moon. Before and after totality, and for partial and annular eclipses, proper eye protection is essential.
- Pinhole Projectors:
- Simple pinhole projectors can be used to safely view a solar eclipse indirectly by projecting the Sun’s image onto a surface.
- Telescope and Binoculars:
- Telescopes and binoculars can provide a closer view of the eclipse but require special solar filters to protect your eyes.
Cultural and Scientific Significance
Solar eclipses have been significant throughout history:
- Cultural Impact:
- Historically, solar eclipses were seen as omens or divine messages in many cultures. They inspired myths, stories, and religious ceremonies.
- Scientific Studies:
- Eclipses have contributed to scientific discoveries. For instance, observations during the total solar eclipse of 1919 provided evidence supporting Einstein’s theory of general relativity by showing the bending of starlight by the Sun’s gravity.
Upcoming Eclipses
Solar eclipses occur about 2-5 times a year, but total eclipses at any given location are rare, occurring roughly once every 360 years on average. Tracking upcoming eclipses allows enthusiasts and scientists to prepare for these events.
Conclusion
Solar eclipses are remarkable events that provide a unique opportunity to witness the dynamic relationship between the Earth, Moon, and Sun. Whether through direct observation with the right safety measures or via cultural and scientific lenses, these celestial events continue to captivate and inspire humanity.
Solar Activity and Space Weather
The Sun exhibits various forms of activity, including solar flares and coronal mass ejections (CMEs), which can have significant impacts on space weather. Solar flares are sudden bursts of radiation, while CMEs involve massive clouds of solar plasma being hurled into space. These phenomena can disrupt satellite communications, navigation systems, and even power grids on Earth. Understanding and predicting solar activity is thus vital for mitigating its potential impacts on our technology-dependent society.
The Sun in Culture and Science
Throughout history, the Sun has held a central place in many cultures and religions. Ancient civilizations worshiped it as a deity, and its movements were meticulously recorded to create calendars and agricultural schedules. In modern times, the study of the Sun has advanced significantly with the help of technology. Solar observatories and space missions, such as NASA’s Parker Solar Probe, have provided unprecedented insights into the Sun’s structure, behavior, and influence on the solar system.
Proxima Centauri: Our Nearest Star
Introduction
The nearest star to our Solar System is Proxima Centauri, part of the Alpha Centauri star system, located approximately 4.24 light-years away from Earth. Proxima Centauri, a red dwarf star, is of particular interest to astronomers due to its proximity and potential for hosting habitable planets.
Characteristics of Proxima Centauri
Proxima Centauri is a red dwarf star, classified as an M-type star. Here are some key characteristics:
- Size and Mass:
- Proxima Centauri has a radius about 14% that of the Sun and a mass about 12% of the Sun’s mass.
- Luminosity:
- It is significantly less luminous than the Sun, with a luminosity of only about 0.17% that of the Sun. This makes it invisible to the naked eye from Earth.
- Surface Temperature:
- The surface temperature of Proxima Centauri is approximately 3,042 degrees Celsius (5,507 degrees Fahrenheit), much cooler than the Sun’s surface.
- Magnetic Activity:
- Proxima Centauri exhibits significant magnetic activity, including flares that can increase its brightness temporarily. These flares result from magnetic field interactions in the star’s convective zone.
The Alpha Centauri System
Proxima Centauri is part of a triple star system known as Alpha Centauri, which includes:
- Alpha Centauri A (Rigil Kentaurus):
- A G-type main-sequence star similar to the Sun, though slightly larger and more luminous.
- Alpha Centauri B (Toliman):
- A K-type main-sequence star, smaller and cooler than the Sun.
- Proxima Centauri:
- The closest of the three to our Solar System, orbiting the pair of Alpha Centauri A and B at a distance of about 0.21 light-years.
Proxima Centauri’s Planetary System
Proxima Centauri has at least two confirmed exoplanets:
- Proxima Centauri b:
- This exoplanet orbits within the star’s habitable zone, where conditions might be right for liquid water to exist. It has a minimum mass of about 1.17 Earth masses and orbits the star every 11.2 Earth days. Due to its proximity to the star, Proxima b is subject to intense stellar flares, which could affect its habitability.
- Proxima Centauri c:
- A larger planet, likely a super-Earth or mini-Neptune, with an orbital period of around 5.2 Earth years. Its distance from the star makes it less likely to be within the habitable zone.
Importance of Studying Proxima Centauri
Proxima Centauri’s proximity makes it a prime target for astronomical studies and potential future space missions. Understanding this star and its planetary system can provide insights into:
- Stellar Evolution:
- Studying Proxima Centauri helps astronomers understand the behavior and lifecycle of red dwarf stars, which are the most common type of star in the Milky Way.
- Exoplanet Habitability:
- Observations of Proxima Centauri b provide crucial data on the conditions necessary for habitability and the potential for life beyond our solar system.
- Space Missions:
- Proxima Centauri is a key target for proposed interstellar missions, such as Breakthrough Starshot, which aims to send small, lightweight probes to the star system to gather data.
Conclusion
Proxima Centauri, our nearest stellar neighbor, offers a unique opportunity to study a red dwarf star and its planets up close. As we continue to explore and understand this star system, it may unlock answers to fundamental questions about the universe and the potential for life beyond Earth.
Conclusion
The Sun, our nearest star, is a magnificent and complex entity that sustains life and influences our planet in countless ways. By continuing to study the Sun, we not only deepen our understanding of stellar phenomena but also enhance our ability to protect and optimize life on Earth. The Sun remains a constant reminder of the dynamic and interconnected nature of the cosmos.
Frequently Asked Questions (FAQ) about the Sun
1. What is the Sun?
The Sun is a G-type main-sequence star (G2V) located at the center of our solar system. It is a massive, glowing sphere of hot plasma that provides the energy necessary for life on Earth.
2. How old is the Sun?
The Sun is approximately 4.6 billion years old. This age has been determined through radiometric dating of the oldest meteorites and models of stellar evolution.
3. What is the Sun made of?
The Sun is primarily composed of hydrogen (~74%) and helium (~24%), with trace amounts of heavier elements such as oxygen, carbon, neon, and iron.
4. How big is the Sun?
The Sun has a diameter of about 1.39 million kilometers (864,000 miles), making it about 109 times wider than Earth. It has a mass approximately 333,000 times that of Earth.
5. How hot is the Sun?
- Core Temperature: About 15 million degrees Celsius (27 million degrees Fahrenheit).
- Surface Temperature: About 5,500 degrees Celsius (9,932 degrees Fahrenheit).
- Corona Temperature: Up to several million degrees Celsius.
6. How does the Sun produce energy?
The Sun produces energy through nuclear fusion in its core. During this process, hydrogen nuclei fuse to form helium, releasing vast amounts of energy in the form of light and heat.
7. What is the Sun’s structure?
The Sun’s structure includes:
- Core: The innermost region where nuclear fusion occurs.
- Radiative Zone: Energy is transferred outward by radiation.
- Convective Zone: Energy is transferred by convection currents.
- Photosphere: The visible surface of the Sun.
- Chromosphere: The layer above the photosphere, visible during solar eclipses.
- Corona: The outermost layer, extending millions of kilometers into space.
8. Does the Sun rotate?
Yes, the Sun rotates, but not uniformly. The equator rotates approximately every 25 days, while the poles rotate about every 35 days. This differential rotation is due to the Sun’s gaseous nature.
9. What are sunspots?
Sunspots are temporary regions on the Sun’s surface that appear darker because they are cooler than the surrounding areas. They are caused by magnetic activity and are often associated with solar flares and other phenomena.
10. What are solar flares and coronal mass ejections (CMEs)?
- Solar Flares: Sudden, intense bursts of radiation caused by the release of magnetic energy.
- Coronal Mass Ejections (CMEs): Massive bursts of solar wind and magnetic fields rising above the corona and being released into space.
11. What is the solar cycle?
The solar cycle is an approximately 11-year cycle of solar activity, marked by variations in the number of sunspots, solar flares, and CMEs. The cycle includes periods of solar maximum (high activity) and solar minimum (low activity).
12. How far is the Sun from Earth?
The Sun is approximately 149.6 million kilometers (93 million miles) from Earth, which is also known as 1 Astronomical Unit (AU).
13. What will happen to the Sun in the future?
In about 5 billion years, the Sun will exhaust its hydrogen fuel and enter the red giant phase, expanding and eventually shedding its outer layers. The remaining core will collapse into a white dwarf, surrounded by a planetary nebula.
14. Why is the Sun important?
The Sun is the primary source of energy for Earth, driving climate, weather, and the photosynthetic process essential for life. Its gravitational pull keeps the solar system in orbit.
15. Can we see the Sun’s interior?
We cannot see the Sun’s interior directly, but scientists study it using helioseismology, which analyzes oscillations and waves on the Sun’s surface to infer details about its inner structure.
16. What is the solar wind?
The solar wind is a stream of charged particles (mainly electrons and protons) released from the upper atmosphere of the Sun, the corona. It extends throughout the solar system and can affect the Earth’s magnetosphere, causing phenomena like the auroras.
17. What is an eclipse?
An eclipse occurs when one celestial body moves into the shadow of another. A solar eclipse happens when the Moon passes between the Earth and the Sun, temporarily blocking the Sun’s light. A lunar eclipse occurs when the Earth passes between the Sun and the Moon, casting a shadow on the Moon.
18. How does the Sun affect Earth’s climate?
The Sun is the primary driver of Earth’s climate and weather systems. Variations in solar radiation can influence climate patterns, though the current climate change is primarily driven by human activities.
19. What are prominences?
Solar prominences are large, bright features extending outward from the Sun’s surface, often in loop shapes. They are anchored to the Sun’s surface in the photosphere and extend into the corona, consisting of cool, dense plasma compared to the surrounding corona.
20. How do we study the Sun?
Scientists study the Sun using various methods, including ground-based telescopes, space-based observatories like the Solar and Heliospheric Observatory (SOHO), and missions like the Parker Solar Probe, which approaches closer to the Sun than any previous spacecraft.
21. What are solar neutrinos?
Solar neutrinos are elementary particles produced during the nuclear fusion reactions in the Sun’s core. They are incredibly difficult to detect due to their weak interactions with matter, but studying them provides valuable information about the fusion processes happening in the Sun.
22. What is the Sun’s role in the Milky Way galaxy?
The Sun is a relatively ordinary star located in the Milky Way galaxy’s Orion Arm. It orbits the center of the galaxy at a distance of about 26,000 light-years, completing one orbit approximately every 225-250 million years.
23. Can the Sun produce a supernova?
No, the Sun cannot produce a supernova. Supernovae occur in stars much more massive than the Sun. Instead, the Sun will eventually become a red giant and then a white dwarf.
24. How does the Sun’s magnetic field affect the solar system?
The Sun’s magnetic field extends far into space and influences the entire solar system, creating the heliosphere—a bubble-like region of space dominated by the solar wind. This magnetic field affects planetary magnetospheres, cosmic rays, and interstellar medium interactions.
25. What is the importance of studying solar cycles?
Studying solar cycles helps scientists understand solar activity’s impact on space weather, which can affect satellite operations, communication systems, and power grids on Earth. It also aids in predicting solar flares and CMEs that can pose risks to astronauts and spacecraft.
26. Are there other stars similar to the Sun?
Yes, there are many stars similar to the Sun, known as solar analogs or solar twins. These stars share similar mass, temperature, and spectral characteristics, providing comparative models to study stellar evolution and planetary system formation.
27. What are coronal loops?
Coronal loops are structures of hot plasma within the Sun’s corona, shaped by magnetic field lines. They often occur in active regions where the magnetic field is particularly strong and are visible in ultraviolet and X-ray wavelengths.
28. Why does the Sun appear yellow?
The Sun emits light across all wavelengths, but it appears yellow from Earth due to the scattering of shorter (blue) wavelengths by the atmosphere. In space, the Sun would appear white, as it emits light relatively evenly across the visible spectrum.
29. Can we harness solar energy?
Yes, solar energy can be harnessed using photovoltaic cells (solar panels) that convert sunlight directly into electricity, and solar thermal systems that use sunlight to heat fluids and produce steam to drive turbines for electricity generation.
30. What is space weather?
Space weather refers to the environmental conditions in space influenced by the Sun, including solar wind, magnetic storms, and radiation. It can impact satellite operations, GPS systems, power grids, and astronaut safety.